Tube Amplifiers Explained, Part 8: Output Stage and Pentode Tubes
- Perry
- Mar 29, 2020
- 8 min read
Updated: Apr 4, 2020
Part of a blog series Tube Amplifier Circuits Explained
The tube used in our driver stage is a triode, which has great qualities, but also some limitations. A triode has a small amount of undesirable internal capacitance that exists between the electrodes, notably between the anode and grid, referred to as Miller capacitance. Historically, this caused problems in particular when attempting to use triodes for radio frequencies. To deal with this, new types of tubes were invented. First the tetrode introduced a screen in between the anode and control grid to shield the grid from the anode, reducing this capacitance. The screen could be held at a constant voltage while most electrons would still pass through to the anode.
However (there’s always a however!) to make it work properly, the screen must be held at a relatively high DC voltage (usually lower than the anode), and this introduces a new complication in our expected current flow in the tube. At certain voltages, electrons will hit the anode with high velocity, dislodging extra electrons which bounce back and are absorbed by the high voltage screen instead of the anode. This is a form of emission from the anode, impacting its voltage at certain amplitudes of the signal, causing a bend in the grid curves, and distortion in the amplification process. Not good, eh?
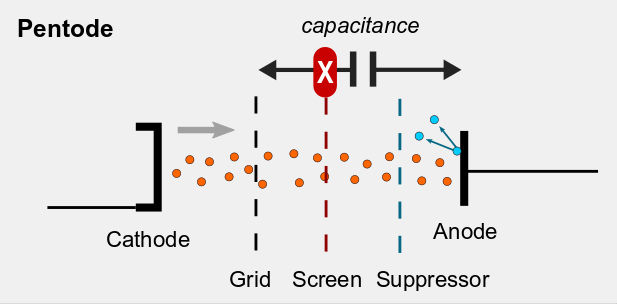
To deal with this side-effect, the pentode was invented, which adds yet another grid called the suppressor in between anode and screen, held at a low voltage (often tied to cathode) and this keeps those bounced electrons from being attracted to the screen, and they are then recollected by the anode. The electrons emitted by the cathode are moving at such a high velocity that the suppressor has minimal effect on them, only the deflected electrons.
The general principle of the power stage pentode tube is similar to the driver triode tube—put high voltage on the anode and screen, put the grid at a voltage that is negative relative to the cathode and modulate it with the voltage signal from the driver stage. But the output tube will be working with higher current and voltage swings.
The pentode screen grid has another effect on the tube, which is that gain is increased, and this is an attractive feature for a power amplification stage. Because the screen is held at a constant voltage, it continuously will attempt to pull a steady flow of electrons from the cathode toward the anode (as much as the control grid will permit), so the tube can conduct current more efficiently. This is different from a triode where the voltage of the anode rising and falling with the signal is the only form of electron draw—for example, as the triode grid voltage moves more positive, current rises causing a drop in anode voltage across the load, but the lower anode voltage is less attractive to electrons than otherwise could be possible. This is a sort of headwind to realizing maximum triode gain that is solved with the pentode.
In our circuit, we are using one type of pentode tube, the EL34. Notice on the schematic the bottom dotted line is the grid, the next one is the screen, and the top one is the suppressor. Note that a pentode tube could be made to operate in triode mode by simply connecting the screen to the anode so they are always at the same voltage. Some amplifier designs do this and we will discuss soon some choices of how we will actually use the screen in ultralinear mode versus pentode or triode mode.
On the schematic, you can see again we have... another grid-leak resistor... and a cathode resistor with bypass capacitor... and an anode load—oh, wait! Where’s the load resistor? The B+ in the output stage is going into the output transformer primary, and out the other side of the primary, to the anode. Aha! We don’t have a resistive load in this case, we have the speaker as the load, and the fluctuating voltage across the transformer is going to drive—or do the work—of moving the speaker coil. But speakers are not designed for high voltage (and it wouldn’t be safe to have this exposed outside of the amplifier anyway), so the output transformer will convert our amplified plate voltage to a different voltage and current level suitable to drive the speakers.
Ok, you are saying, but wasn’t the whole point to amplify the signal? Are we reversing our process? No. Remember that the transformer will transfer the same amount of power from the primary side to the secondary side, but the number of windings in the transformer will determine how current and voltage are changed. In our case, we are lowering voltage down to levels below 10V, but for power to be the same, current must increase. So we will have a large AC current driving our speakers. This is a transformation to the final step of our audio signal and we hope our trusty output transformer does this as a faithful and accurate reproduction. The quality and size of the output transformer is very important in maintaining a flat frequency response across a wide bandwidth at our desired output power. The AE1 kit includes an Edcor brand transformer, made in the USA, that I have used extensively and believe is very high quality for the cost. It is rated to 15 watts of power and with relatively good frequency response. There are smaller and larger sizes of transformer (“iron” as you might say to your DIY tube amp buddies), and larger ones will be heavier and more costly, but also will ensure a wide bandwidth and flat frequency response at higher rated power output. The takeaway is that not all are created equal, so this is one place in your amp where you want to choose carefully a trusted and high quality manufacturer.
So if the speaker is the load on the power tube, how big of a load is it and can we calculate the load line again? This kit amplifier is designed to work with an 8 ohm speaker impedance, but the windings ratio of the transformer will make this impedance look much larger to the tube which is what we need for the tube to operate properly. We select this ratio when choosing the output transformer. Our design will use a transformer that will be 5,000 ohms on the primary side of the transformer for an 8 ohm secondary impedance. You could choose a transformer with different values, depending on the load you want on the tube and the impedance of your speakers. I chose an 8 ohm output impedance for this kit because this is a very commonly available speaker. And 5,000 ohms gives us a load that will work effectively for our supply voltage and characteristics of the EL34 tube.
Speaker impedance
It is important to remember that the speaker is not a uniform resistance of 8 ohms. All speakers have impedance that varies with frequency. They are rated typically at 4 ohms, 8 ohms, 16 ohms, but this is just a nominal value generally close to the impedance that could exist at any given frequency. A typical 8 ohm speaker could actually have a wide range of impedance from 3 or 4 ohms at some frequencies or 10 or 20 ohms or higher at other points. The job of the speaker is to reproduce frequencies as uniformly as possible across the audible frequency spectrum, and there are physical properties to the cone and suspension and a wide range of other things that will cause the impedance to be higher or lower across frequencies.
So if we know the B+ in our circuit is 400V and we have a 5,000 ohm load, we can start to work on a load line and operating point. Like the triode tube in our driver stage, we can look at the tube characteristics provided by the manufacturer. The process will be a bit different because this is a pentode tube and because of our output transformer and speaker load. One challenge is that the load lines will vary depending on the screen voltage. Sometimes the datasheet will have multiple charts for different screen voltages. Below is one for 360V, which is relatively close to our expected voltage that we could put on the screen. It’s ok if this isn’t perfect, we are just getting somewhat close to the theoretical operating point and it becomes important to test and modify the circuit in actual operation to optimize it anyway.
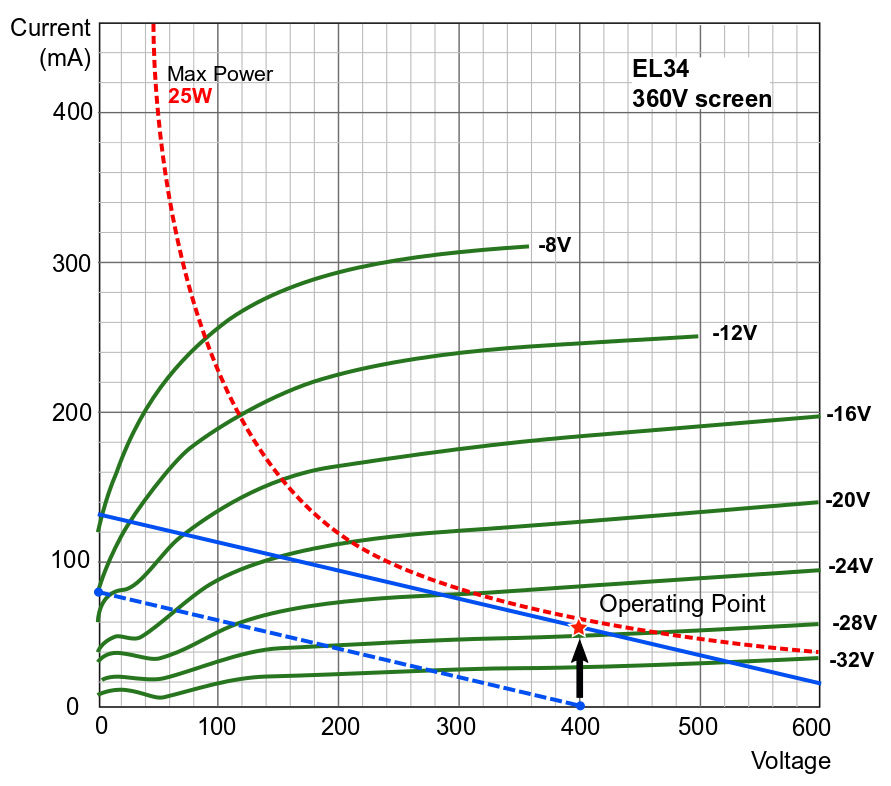
In the chart shown, the blue dotted line is the initial load line for 400V and 5,000 ohms (which would be 80mA of current if all 400V were dropped). But remember that we do not have a resistive load. (Actually, the transformer has a small amount of DC resistance in the primary winding—about 160 ohms for the ones included in this kit—but this is so small we will ignore it.) So at the quiescent point with no AC signal, nearly the entire B+ voltage will be on the anode, so our operating point voltage must be around 400V. But if we stay on our initial load line at this point, it would not be useful because it’s at cutoff and could then only amplify the positive side of the signal. We need to bias the tube and move this load line up.
What we will do is choose an amount of current that we want at the 400V operating point. To maximize our power from this tube, let’s pick a level just below the maximum rating, shown with the red dotted line. Approximately 60mA of current would keep us under the 25W maximum. So we shift our load line up, keeping the same slope until we reach an operating point that is 400V and 60mA. Finding the nearest grid line, this is pretty close to -28V. See that? (For simplicity, let’s just pick ‑28V, though we could try to estimate -27V, etc.) Now, if we need -28V on the grid relative to cathode, then we can again use the cathode bias technique and hold the grid at 0V while we elevate the cathode to +28V. Using Ohm’s law, if we are estimating 60mA of current dropping across 28V, this gives us a resistance of 466 ohms, or let’s pick 470 which is a common resistor value. This will dissipate about 1.7W of power, so we would choose a resistor rated at least double for a margin of safety, such as a 5W resistor. We will again bypass this capacitor with a 100uF electrolytic capacitor, but this time rated higher, such as 100V.
Are you still with me? I know it’s a lot, but you are getting it, right? Summarizing for a moment… we put 400V on the anode and because there is little resistive load under DC conditions, this is our operating point voltage. We elevate the cathode to 28V and our tube will be dissipating 60mA of current in its quiescent state, shifting our load line up. Now under AC conditions the signal will vary the voltage of the grid by let's say 10 volts or so depending on input level and amplification in the driver stage, and this will swing the anode voltage very widely, up to a few hundred volts. And importantly, it will put this alternating current across the primary side of the transformer, where it's converted back down to a low voltage and higher current on the secondary side to drive the speakers. Notice here that the anode voltage can actually rise higher than the B+ supply because the transformer stores energy in its magnetic field and it will resist the change in current, causing it to create a temporary higher voltage.
A few more quick notes. We haven’t illustrated the AC load line in the chart, and will not try to do this because the speaker impedance varies across frequencies. But you could visualize an AC load line pivoting around this operating point, sometimes a higher load and sometimes lower, altering the slope of the line, but always around the operating point. At this time, we know enough about the operating point of the circuit to have our initial design and can modify if needed.
We also need to get back to that screen. You thought I forgot, didn’t you? Take a break, get a snack. Almost there!
Coming next, Screen Voltage and Ultralinear Mode.
Comments